Power
Boosting Converter Economy
Minimising standby current in ‘permanently connected’ automotive systems demands careful and clever power supply design. Matthias Ulmann, Reference Design Engineer in the EMEA Design Services Group at Texas Instruments, explores further in this ES Design magazine article.
In aThis article offers some advise on reducing quiescent current in shutdown mode and gives hints for the design of converters in general and especially boost converters in an automotive environment. Of course, the techniques and ideas shown here can be used also for other applications and different types of converters.
Figure 1 shows the block diagram of a typical automotive power solution. The input of the system is connected directly to the battery. Buck 1 is always enabled and generates a voltage of 3.3V, which supplies a microcontroller amongst other things. If the system is not in use, the microcontroller disables buck 2, 3 and the boost converter before it goes into sleep mode itself. Buck 1 is still active and keeps the microcontroller supplied, but it needs to switch to some kind of pulse skipping mode to reduce the losses.
When the microcontroller receives the signal to wake up, it enables buck 2, 3 and the boost converter. Buck 1 will automatically switch from pulse skipping mode into normal operation mode. This can be discontinuous conduction mode at light load or continuous conduction mode when the nominal current is drawn.

The input voltage can range from 4.5V during cranking to 18V at a load dump; additionally, the converters have to withstand peak voltages of up to 40V. A boost converter is placed in front of the two bucks; as long as the battery voltage is equal or higher than 10.0V it is not switching but when the battery voltage drops below 10.0V, it automatically starts to boost the voltage to 10.0V to supply the two bucks with a voltage high enough to keep the output voltages constant.
This is a simple solution and has the drawback that even when the boost is not switching its output diode is in series to the load, causing a voltage drop and therefore additional losses. There are also other approaches but the system shown in Figure 1 is the most commonly used due to its simplicity and reliability.
As the boost converter doesn’t work continuously, from a thermal perspective only the average losses have to be dissipated. This saves not only space but enables the designer also to use smaller and cheaper parts.
Beyond Automotive
A simplified boost converter is shown Figure 2. It has some additional circuitry which is not only useful for automotive applications. The controller is supplied by an ORing diode (D-OR) either from the input voltage or from the output voltage, depending on which voltage is higher (usually the output voltage). This diode brings two advantages; first, the booster can work on an input voltage which can be lower than specified in the datasheet and, secondly, the gate driver works with a higher voltage than the low input voltage, which reduces the losses of the external FET (Q-SW).
An integrated linear regulator generates a stabilised voltage of 8V to supply the IC and is buffered by an external ceramic capacitor (C-BP). If the input voltage is not high enough to enable the linear regulator, the lower input voltage directly supplies the IC and therefore also the gate driver. This results in lower efficiency as the on-resistance of a FET is higher with a 4.5V gate-source voltage compared to 10V.
The diode should be added to each boost converter if the input voltage can drop below the output voltage of the integrated linear regulator. This results in higher efficiency and lower losses and adds only little additional cost.

The feedback path consists normally of two resistors forming a voltage divider (R-FBH, R-FBL) between the output and ground. This voltage divider is always present, causing an additional current as the output voltage is equal to the input voltage when the booster is switched off. To reduce the current through the divider, an easy solution would be to make it highly resistive. Unfortunately, in automotive applications the maximum resistance is usually limited to 100kΩ. To deal with this problem, a small FET (Q-EN) is placed between the low side resistor and ground. The enable signal is used to connect the resistor divider to ground, to make it functional.
But this causes a new problem; as already noted peaks of up to 40V are seen on the input as well as on the output of the booster, thus it is also seen on the feedback voltage divider and reduced on the feedback pin. If the divider is connected to ground all the time, there is no problem as the low side resistor (R-FBL) is usually much smaller than the high side resistor (R-FBH) and the absolute maximum rating of the feedback pin is 10V on TPS40210. With the low side resistor isolated from ground during shutdown, the full peak voltage is seen on the feedback pin. A zener diode (D-CLAMP) connected from FB to ground clamps the voltage to a reasonable value and protects the IC. The zener voltage should be selected close to the maximum rating of the FB pin (8.2V in this case), as during shutdown mode the battery is still connected and a low current flows always through R-FBH and the clamping diode. That’s also the reason for using the maximum resistance of 100kΩ for the high side resistor of the voltage divider.
If the IC applied has a dedicated input for undervoltage lockout (voltage divider), its maximum voltage rating myst also be checked and, if necessary, a clamping diode added.
The schematic in Figure 2 has an enable input (J3), but it is not directly connected to the enable input of the IC itself (DIS/). Pulling DIS/ low (<0.7V) or leaving it open enables the booster, pulling it high (>1.3V) disables it. The feedback pin is connected to the enable input DIS/ by two diodes in series. If the booster is disabled by input J3, FET Q-EN isolates the low side resistor of the voltage divider R-FBL from ground and the voltage on pin FB is clamped to 8.2V. This voltage is connected to the enable input by two diodes, so it is well above the minimum of 1.3V. If the booster gets the enable signal, the voltage divider is connected to ground causing the voltage on DIS/ to drop below 0.7V. The converter starts working and regulates the output voltage, such that the voltage on the feedback FB equals 700mV. Due to the two diodes in series this voltage is not seen on pin DIS/ and the integrated pull-down resistor keeps DIS/ at ground potential.
FET Control
Figure 3 shows another example of how to control the FET of the feedback voltage divider. Unlike in Figure 2, the enable signal does not directly drive the FET. The gate is connected to the output of the integrated linear regulator on pin VCC. When the converter is enabled and therefore the linear regulator inside the LM3481, it automatically switches on the feedback voltage divider. This happens very fast compared to the ramping of the output voltage, without causing any overshoot on the output. A pull-down resistor and a small capacitor are placed directly between gate and source, and keep it low safely as long as no voltage is applied.

The enable input of LM3481 is kind of special as it has multiple functions. First, with a resistor connected to ground, the free-running switching frequency is set. Second, it is the input for the synchronisation signal if the converter’s switching frequency has to be synchronised to other converters. And third, the converter enters shutdown mode if a voltage of 1.4V or higher is applied to this pin.
In this example, an LDO TPS7A1633 with high input voltage range (up to 60V) and low quiescent current (5μA) is connected directly between the battery and the enable pin. If the LDO is enabled, the 3.3V output voltage is applied to this pin forcing the boost controller into shutdown. Additionally, the 3.3V output can be used to power other circuitry which needs to be active during shutdown of the system, for example the microcontroller in Figure 1. This can be more efficient than using Buck 1 to generate the supply voltage for the microcontroller during shutdown of the system. To start the booster, the LDO is disabled and its output becomes high impedence. Now, only the resistor R-FA which sets the switching frequency is electrically connected to this pin.
The examples and design tips shown can clearly improve the overall efficiency and performance of boost converters in automotive environments. The additional circuitry is very small, but the improvement significant. Therefore its use is not limited to this application, it basically helps to save power and lower the quiescent current in all other environments.
When starting with the power supply system design, care has to be taken on the specified voltage spikes and on which parts of the system they may appear. The right part selection and, if needed, clamping circuits avoid any problems.
Author Profile: Matthias Ulmann was born in Ulm, Germany, in 1980. He was awarded a degree in electrical engineering from the University of Ulm in 2006. After working for several years in the field of motor control and solar inverters (specialising in IGBT-drivers), he joined TIs’ Analog Academy for a one year trainee program. Since 2010 he has worked in the EMEA Design Services Group as a Reference Design Engineer in Freising, Germany. His design activity includes isolated and non-isolated DC-DC converters for all application segments.
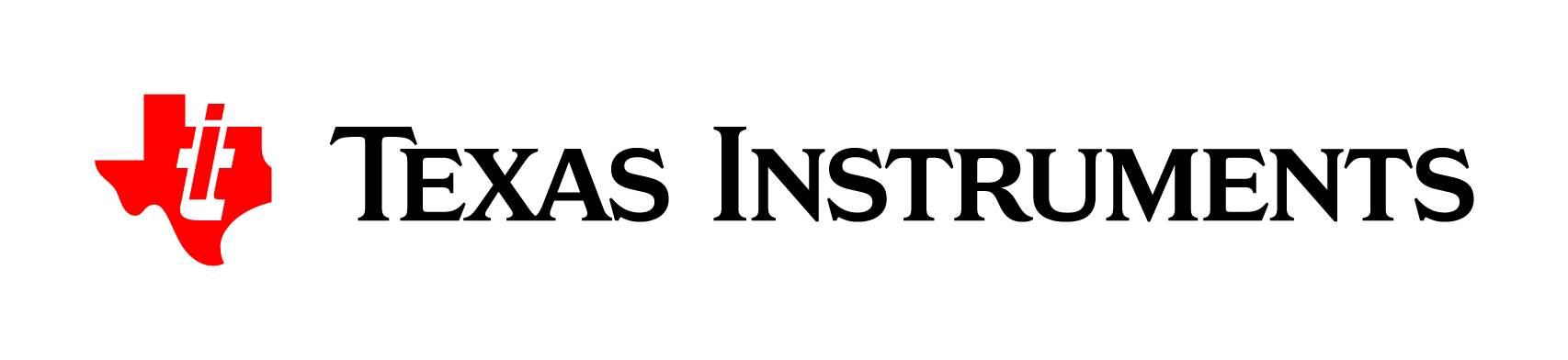