Optimising automotive pre-boost power supplies - part 3
Part one of this article described the problem, showed the impact of a cold start test pulse on an automotive power supply and discussed a typical pre-booster specification. The second part provided guidance on proper part selection of all relevant components. This third and final part demonstrates the differences between operation modes and the influence of the inductance and capacitance on performance. By Matthias Ulmann, Texas Instruments.
Mode of operation
If a boost converter is working in discontinuous conduction mode (DCCM, inductor current goes to zero during each switching cycle), the right half plane zero is at such a high frequency that it basically has no impact on the maximum bandwidth of the converter. In this mode of operation, the achievable bandwidth is in the range of 10-20kHz, about one decade higher than CCM. Regarding the compensation network, the resistor becomes bigger and the capacitors smaller meaning they can be charged much faster by the error amplifier and at the end, the voltage breakdown on the output will be significantly lower. Table 2 shows the test result of a booster working in CCM and DCCM. The compensation network of the booster working in DCCM was designed so that it has at least 60 degree phase margin under all conditions like the one working in CCM.
Table 2 - CCM vs. DCCM
Going from CCM to DCCM improves the output voltage regulation even further than just doubling the bandwidth as shown before. The breakdown of the output voltage is now only 12%, but the maximum inductor current is 50% higher. When the inductor current falls to zero during each switching cycle and the power remains the same, the peak current becomes naturally higher. This has to be kept in mind when selecting the input and output capacitors, because the ripple current stress becomes much higher, especially on the input. Also regarding EMI, DCCM operation is potentially harder to handle due to the larger peak currents.
It is important to note, that the soft-start feature of the controller has no impact on any delay seen here. The soft-start is only active at the very first start-up of the controller. In this application, the controller is already running, but not switching. So when the output voltage falls below the programmed level, no soft-start is active and only the error amplifier limits the duty cycle.
Impact of the inductance
For all the following measurements related to the passive components of the power stage, the compensation has been adjusted so that the bandwidth is nearly the same, to highlight only the influence of the passive components. The phase margin is at least 60° and the gain margin at least -20dB.
As shown in the previous section, the inductance has a large impact on the maximum bandwidth as it sets the mode of operation (CCM vs. DCCM). If the converter should work in CCM, the possible inductance range is quite large. But does the inductance have an influence on the output voltage regulation as well?
The equation above shows, that the slew rate of the current ∆i/∆t of an inductor depends on the inductance and the voltage applied. If the voltage is constant, the rate of current change depends only on the inductance. So in this context, the inductance could have an influence on the voltage regulation. Table 3 shows a comparison with a different inductance from the standard test setup.
Table 3 - 76 vs. 35% current ripple
No difference regarding output voltage regulation is measurable if the current ripple is decreased from 76% down to 35%. In summary, the inductance and therefore the inductor’s ∆i/∆t of a booster working in CCM has no influence on the breakdown of the output voltage. But, as shown previously, the inductance should be selected as low as possible to shift the right half plane zero to higher frequencies for maximising the achievable bandwidth.
Impact of the input and output capacitance
The input capacitance buffers the booster’s input voltage and reduces the falling slope when the test pulse is applied. This dampens the effect of the slow start-up of the booster due to saturation of the error amplifier’s output and improves output voltage regulation. Table 4 illustrates this effect if the input capacitance is varied.
Table 4 - 220 vs. 440µF input capacitance
The second comparison (Table 5) shows the improvement if the output capacitance is increased.
Table 5 - 47 vs. 147µF output capacitance
In summary, increasing the output capacitance by 100µF has a larger impact than increasing the input capacitance by 220µF. A look at the voltage applied to these capacitors clarifies this result. When the test pulse is applied and the input voltage is falling, the input capacitor is discharged by the booster, which is starting up but not recharged by the input voltage anymore. Due to discharging the input capacitors, voltage decreases, which causes the current to rise and the capacitor to be discharged even faster. The output capacitor has to buffer the voltage just until the booster is starting up. Then it is recharged to the nominal output voltage and the load current is supplied fully by the booster. With a larger output capacitance, the maximum inductor current is slightly higher as more energy is needed for recharging.
“Jumping the gun”
Independent of the optimisation of the power stage, the primary reason for the breakdown of the output voltage on a test pulse is the delay caused by the error amplifier’s saturation. Reducing this delay would bring a bigger improvement than all other possibilities shown. This is possible if the pre-booster is already switching before the input voltage descends. Therefore the output voltage needs to be set to a voltage which is always higher than the maximum battery voltage, for example 15.0V. With an additional small FET and resistor this functionality can be added easily, as displayed in Figure 6.
Figure 6 - VOUT adjustment
While the FET is open, only the resistor which sets the output voltage to 9.0V is active. This is the normal operation mode when the engine is either running or not and the battery voltage is higher than 9.0V. If the car is parked for longer periods, for example at an airport, the battery voltage decreases slowly. If it falls below 9.0V, the booster starts automatically and maintains a stable output voltage for the system. This is a very slow process and no special optimisation, like for a cranking event, is needed. The automatic start-up of the pre-booster is only desired for a few systems that have to be always active, for example keyless entry or immobiliser. Most of the systems should be switched off to reduce the drain of the battery. So depending on the application, the pre-booster needs to be disabled when the car is parked to avoid an automatic start-up.
Now, just before the engine is started, the FET is switched on and adds the second resistor in parallel. As the boost controller is supplied all the time, it immediately starts switching without any soft-start delay and sets the output voltage to 15.0V. Next, the engine is started and since the booster is running, it can react immediately without any delay when the input voltage drops. This approach is only possible as in modern cars the starter motor is not controlled directly by the key anymore but by an MCU. The status of the key switch or push-button is known by the system and according to that the starter motor is activated. Starting the pre-booster before the engine takes only a few tenths of milliseconds and will not be noticed by the driver.
Table 6 - 9.0 vs. 15.0V output voltage
The results in Table 6 highlight the advantage of starting the pre-booster before the test pulse is applied. If the output voltage is set to 9.0V, the output voltage drops around 58% down to 3.8V. This is even lower than the output voltage of the subsequent buck converters and will lead to the electronic system shutting down. With the booster set to 15.0V, a voltage drop of only 15% down to 12.7V is visible at the output. This voltage is well above the buck’s output voltages and the system will work during the test pulse without any interruption. Additionally, the maximum inductor current is slightly lower.
The breakdown of the output voltage could be even higher; down to 7.0V would be no problem for the 5.0V buck converter. Thus, the input and output capacitance can be reduced even more, which saves space on the PCB and is more cost-effective. Similar designs using this approach incorporated only ceramic capacitors on the input (1x4.7µF) as well as on the output (1x33µF), which resulted in a very tiny solution that is still able to provide superior performance.
Conclusion
Designing a proper pre-booster design with good performance requires some background knowledge. This article describes in detail the impact and optimisation of all components, including the controller and the power stage. The “traditional” approach of a pre-booster just waiting until the output voltage breaks down and then starting to switch does not produce the best results. However, it can still have satisfying performance, if the power stage is well designed and selected. Moving from CCM to DCCM boosts performance at the price of higher peak currents, which is a concern especially for the input and output capacitors. Nowadays, the best approach is to activate the booster with a higher voltage than the battery voltage before the starter motor is started. Then the booster is already switching and reacts on the decreasing input voltage without any delay. Not only does this minimise the output voltage drop more than any other approach, it also offers the possibility to reduce the input and the output capacitance significantly. If this is not possible, Texas Instruments TPS4333x pre-booster + dual buck controller family is the best choice. The pre-booster auto-activates at approximately 1V above the set output voltage and provides a well-regulated voltage for the synchronous bucks.
All measurements were done using TI’s inexpensive and handy cranking simulator, which can be found on ti.com with reference PMP7233 and AutoCrankSim-EVM.
About the author
Matthias Ulmann was born in Ulm, Germany, in 1980. He was awarded a degree in electrical engineering from the University of Ulm in 2006. After working for several years in the field of motor control and solar inverters (specialised in IGBT-drivers), he joined TIs’ Analog Academy for a one year trainee programme. Since 2010 he has worked in the EMEA Design Services Group as a Reference Design Engineer in Freising, Germany. His design activity includes isolated and non-isolated DC/DC converters for all application segments. He was elected “Member, Group Technical Staff” in 2015.
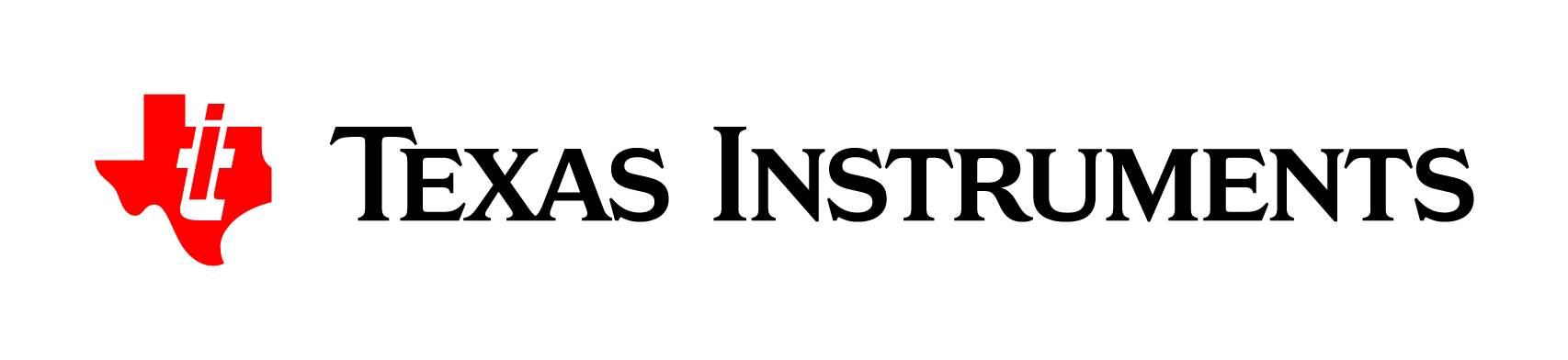